Welcome to the OES Standing Committee on Standards
The OES Standing Committee on Standards aims at promoting the development and widespread use of standards and interoperability of collected data in the domain of ocean science and technology. By that, ocean observations can be assigned agreed upon quality levels and ensure more efficient and sustainable use of ocean data and information. With OES having strong links to manufacturers of ocean instruments the competitive advantages of products following accepted standards will be discussed and adequate future strategies developed. The involvement of students into standard activities will be ensured by organizing dedicated workshops & symposia.
OES is striving to closely coordinate standardization activities with IEEE SA – The IEEE Standards Association (https://standards.ieee.org). Links to other standardization organizations like OGC and ISO also exist.
Why Standards
Standards serves the purpose to
- ensure that products and services are comparable in their functional scope and that they had been qualified in a similar manner. As an example, manufacturers can only claim the same accuracy for their instrument when they had been tested with equivalent methods
- facilitate interoperability and interchangeability of product and services. This will be necessary if ocean observations in different parts of the world shall be made compatible
- overcome disparate operating procedures that often can be observed within different ocean observing networks
Therefore, standards are so important and useful. However, with all introduced standards there is a certain overhead and cost involved to adopting standards, and the benefits have to outweigh those costs.
By no means the issue of a standard that’s inadequate or quickly outdated will be neglected in the anticipated discussions.
Being the Point of Contact for ocean engineering standards
The OES Standing Committee on Standards is an international coordinating committee of experts with a vested interest in expanding the use of proven standards covering all hardware and software aspects of the data collection and dissemination aspect. The Committee will have a close exchange with those OES members who are spearheading the Ocean Best Practices System (https://www.oceanbestpractices.org) as Best Practices are seen as an intermediate step to establish new standards.
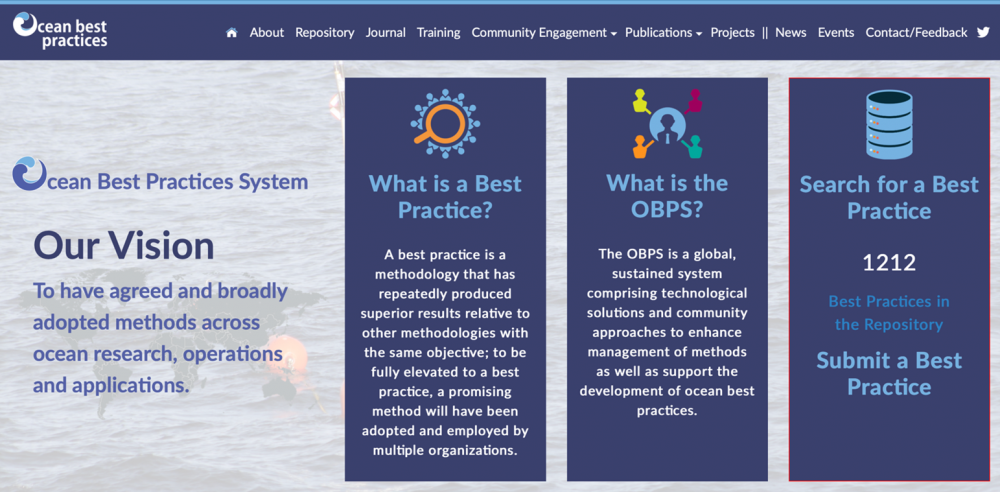

As an example for an expressed need for standards in the domain of ocean engineering ROVs shall be mentioned. They form the backbones for underwater intervention operations and the need for exchanging best practices and following standard procedures soon became obvious.
There are a couple of other ROV standards already existing in industry
and others published by ISO
https://www.iso.org/obp/ui/#iso:std:iso:13628:-8:ed-1:v1:en.
Within underwater digital acoustic communication there is the JANUS Standard although even if it’s a NATO standard it’s free and open to everyone to use (completely public)
http://januswiki.org/tiki-index.php
Ongoing Activities
OES Standards Committee will maintain a dialog between other IEEE Societies and Councils on issues related to standards. When a new one comes out it will be announced through the OES webpage, to inform those other societies and councils who might have an interest be notified of it.
Some examples of ocean science related standards:
https://www.iso.org/standard/72218.html
https://www.ogc.org/standards/sensorthings
https://www.ogc.org/projects/groups/marinedwg
https://www.ogc.org/projects/groups/uxsdwg
Ocean Systems Engineering
The field of Ocean Engineering embraces the practices of formal Systems Engineering as part of its process. As such, the IEEE Ocean Engineering Society Standards Committee cooperates closely with the IEEE Systems Council Standards Committee on matters related to Ocean Systems Engineering. The IEEE Systems Council Standards Committee does not produce new standards related to the discipline of systems engineering since this field is involved more with processes and methodologies than with a set of specific standards per se. However, these two IEEE Standards Committees can recommend, as guidance, specific documents, texts, guidelines, etc. related to employing systems engineering principles which are commonly accepted in academia, industry, and government from various countries. A list of examples follows on their website at: https://ieeesystemscouncil.org/standards.
Additional Oceanic Standards
Keywords: Calibration protocols, quality assurance, best practices, international standards, OES Standards Initiative
Scope: The Standards Committee promotes and participates in the development of standards for use in oceanic engineering. It conducts a Standards Initiative, which aims to compile and disseminate information on standards, protocols, quality assurance procedures, and best practices that are important in oceanic engineering. It does this through the OES website, where information and references are given to standards concerning, thus far, oceanographic data, the offshore oil and gas industry, and underwater acoustics. It sponsors occasional special initiatives, as in the case of the OES Interoperability Standards Initiative for the Marine Environment. The committee also participates in the formulation of standards by the IEEE Standards Association (IEEE-SA), International Organization for Standardization (ISO), International Electrotechnical Commission (IEC), and American National Standards Institute (ANSI). It routinely convenes and conducts sessions at OCEANS Conferences, vets publications on standards, and liaises with other TCs.
Standards Activities
Overview
IEEE member technical societies generally conduct or participate in standards activities commensurate with their discipline. The Oceanic Engineering Society (OES) is pursuing standards in several ways. It is sponsoring a standards initiative, which aims to compile and disseminate information on standards, protocols, quality assurance (QA) procedures, and best practices that are important in ocean engineering. It is also sponsoring an interoperability standards initiative, which aims to define the need for interoperability with respect to sensors used in systems in the marine environment. Both of these initiatives are described in the following webpages.
The OES participates in such standards organizations as the International Electrotechnical Commission (IEC), International Organization for Standardization (ISO), American National Standards Institute (ANSI), and British Standards Institute (BSI), among others.
OES Standards Initiative
The OES Standards Initiative aims to compile and disseminate information on standards, protocols, quality assurance (QA) procedures, and best practices that are important in ocean engineering. The OES Standards Committee is coordinating this work, as well as promoting collaboration with the other Technology Committees of OES. The Committee is also soliciting information on standards from the ocean engineering and oceanographic communities.
General Introduction to Standards
Every industrial country requires a sound infrastructure for measurement. This is reflected in the increasing importance given to quality schemes and accreditation, for example the ISO9000 series. Properly authenticated measurements are required in support of regulation (for example, for environmental assessment and for health care), and to underpin good product design and efficient manufacturing in demanding and competitive markets. However, measurements are only meaningful if they are performed in a technically sound manner and if they can be related to common standards of measurement. The general requirements for a “good-quality” measurement include:
- expertise in the use of the instrument (including any necessary training/certification);
- a calibrated instrument or artefact (with the calibration traceable to agreed standards);
- a standard method and procedure for using the instrument.
The first of these relates to the knowledge, experience and training of the individual undertaking the measurement, which inevitably influence the ability to obtain high-quality results. However, to ensure different individuals consistently obtain comparable results requires a calibrated instrument and an agreed procedure, and it is these two influences that are the subjects of this introduction. In the measurement context considered here, the word “standard” is used with two meanings. Firstly, it refers to a calibration standard – a method (or occasionally an artefact, as is the case for the kilogram) which is used to provide traceability back to a common benchmark. Secondly, it may refer to a specification standard – a written procedure describing the method for undertaking a measurement. Both of these are discussed here.
I. Calibration standards
In most industrialized countries, calibration standards are provided through a hierarchical infrastructure, at the head of which are the primary national standards realized and maintained by national metrology institutes (NMIs). The National Institute for Science and Technology (NIST) in the USA, and the National Physical Laboratory (NPL) in the UK, are examples of such NMIs. Traceability and uncertainty Measurements should ideally be traceable to the standards held at the NMI. This concept of traceability of measurements can be defined as [1]: the property of a result of a measurement whereby it can be related to appropriate standards, generally international or national standards, through an unbroken series of comparisons. Traceability requires that the instruments used for measurement (for example, the hydrophones used in acoustic measurements) be calibrated such that the calibration may be traced back to national standards, either by a calibration undertaken by the NMI, or by an accredited laboratory whose standards are themselves traceable. When comparing two measurements (or comparing one measurement with a required tolerance or specification), it is important to take full account of the measurement accuracy. When making a measurement, it is not generally possible to identify the absolute error since the true value, of the quantity being measured, is not known. Instead, an estimate is made of the uncertainty in the measurement. The uncertainty may be defined as: an estimate of the range of values within which the true value is considered to lie to a specified degree of confidence (for example, for a confidence level of 95%). Analysis of uncertainty is a well-established discipline with standard procedures, such as the international Guide to the Expression of Uncertainty in Measurement [2], for example. Note that uncertainty estimation should be distinguished from the natural variation in the quantity being measured that might occur due to the quantity itself varying. For example, the level of underwater ambient noise will vary with sea-state, and the noise radiated by a vessel may vary depending on the speed and engine loading. There are two general classes of uncertainty, categorised in terms of how they are estimated. Type A uncertainty is sometimes described as the “random uncertainty” or degree of repeatability, and may be assessed by making related measurements of a quantity and examining the statistical spread in the results. However, it may not be possible to make repeated measurements if the event being measured is unique. The Type A uncertainty is a measure of the precision in the measurement – high precision is obtained if the measurements are repeatable with little dispersion in results. The second category is the Type B uncertainty, which is sometimes referred to as the “systematic uncertainty”, and represents the potential for systematic bias in a measurement (caused by incorrect instrument calibration, for example). This category of uncertainty cannot be assessed using repeated measurements, and must be evaluated by consideration of the potential influencing factors on the measurement accuracy. Validation of calibration standards Whilst traceability of measurements to primary standards provides some quality assurance, there remains the question of how to determine that the primary standard itself is accurate. Since it provides the benchmark for secondary and tertiary calibrations, the primary standard cannot simply be compared to a method that is lower down the standards hierarchy. Instead, an assessment of the absolute accuracy must be made, which can be achieved in three ways:
- systematic study of the uncertainties in the primary standard calibration method. This may involve performing experiments to determine the size of the uncertainty contributions;
- where possible, a comparison is made with another independent absolute calibration method, preferably one based on a different physical principle (and therefore with few common sources of uncertainty);
- comparisons are conducted with the NMIs in different countries. Such comparisons help to harmonise standards across national boundaries and can lead to the discovery of previously unknown sources of error.
The result of these comparisons may be expressed in terms of the degree of equivalence between the different countries along with the uncertainties e.g. [3]. This type of exercise provides greater confidence in the individual results and allows for mutual recognition of calibrations undertaken in different countries.
II. Specification standards
Specification standards are documents describing procedures to be followed when undertaking measurements. The highest of such standards are international standards produced under the auspices of organisations such as ISO (International Organization for Standardization) and IEC (International Electrotechnical Commission), for example. ISO produces standards which cover physical measurements; with regard to underwater acoustics this includes environmental noise or noise radiated from specific sources such as ships. IEC produces standards that cover electrical measurements, and this includes the calibration of instruments, such as hydrophones. ISO and IEC standards are typically adopted as national standards within member countries. Sometimes, national standards bodies within individual countries produce their own standards, for example the American National Standards Institute (ANSI) in the USA [4], and the British Standards Institute (BSI) in the UK. In addition, informal guidelines and good practice guides may be produced, which are very useful in promoting good practice, although they do not have the status of international or national standards.
III. Joint standards
There are several other standards which are of relevance to measurement in general, and which are joint publications by several standards bodies including ISO and IEC. These are: JCGM 200:2012, International vocabulary of metrology – Basic and general concepts and associated terms (VIM), 3rd edition. JCGM 100:2008, Evaluation of measurement data – Guide to the Expression of Uncertainty in Measurement (GUM). Both are available from www.bipm.org.
References
[1] JCGM 200:2012, International vocabulary of metrology – Basic and general concepts and associated terms (VIM), 3rd edition, joint publicationby BIPM, IEC, IFCC, ILAC, ISO, IUPAC, IUPAP and OIML, 2012, Available from www.bipm.org [2] JCGM 100:2008, Evaluation of measurement data – Guide to the Expression of Uncertainty in Measurement (GUM), joint publication by BIPM, IEC, IFCC, ILAC, ISO, IUPAC, IUPAP and OIML, 2008. Available from www.bipm.org [3] S. P. Robinson, P. M. Harris, J. Ablitt, G. Hayman, A. Thompson, A. Lee van Buren, J. F. Zalesack, R. M. Drake, A. E. Isaev, A. M. Enyakov, C. Purcell, Z. Houqing, W. Yuebing, Z. Yue, P. Botha, D. Krüger, “An international key comparison of free-field hydrophone calibrations in the frequency range 1 to 500kHz”, J. Acoust. Soc. Am., 120, 1366-1373, 2006. [4] S. B. Blaeser, “International standards development and the U.S. technical advisory group process”, Acoustics Today, 8(1), January 2012.
Oceanographic Data
Equation of State of Seawater
The equation of state of seawater is a function from which the thermodynamic properties of seawater can be derived mathematically. A Gibbs function for this has been developed by Working Group 127 “Thermodynamic and Equation of State of Seawater” as a collaboration of the Scientific Committee on Oceanic Research (SCOR) and International Association of the Physical Sciences of the Oceans (IAPSO). The particular Gibbs function, or Gibbs potential, is a function of absolute salinity, temperature, and pressure. This equation is presented in the following work together with statements of basic thermodynamic properties and such derived properties as potential temperature, potential enthalpy, conservative temperature, potential density, among others.
IOC, SCOR and IAPSO, “The international thermodynamic equation of seawater – 2010: Calculation and use of thermodynamic properties,” Intergovernmental Oceanographic Commission, Manuals and Guides No. 56, UNESCO (English), 196 pp.
This is available for free download at http://www.teos-10.org/pubs/TEOS-10_Manual.pdf (last viewed 29 July 2013).
Two associated documents of potential interest are:
“Getting started with TEOS-10 and the Gibbs Seawater (GSW) Oceanographic Toolbox,” Version 3.0, May 2011, available at http://www.teos-10.org/pubs/Getting_Started.pdf (last viewed 29 July 2013).
A primer: R. Pawlowicz, “What every oceanographer needs to know about TEOS-10 (The TEOS-10 Primer)” is available at http://www.teos-10.org/pubs/TEOS-10_Primer.pdf (last viewed 29 July 2013).
The equation was adopted by the Intergovernmental Oceanographic Commission in June 2009, replacing a series of algorithm dating from 1978. These historical references include the Practical Salinity Scale (PSS-78) and the International Equation of State of Seawater (UNESCO 1981) as well as other algorithms for the specific heat capacity of seawater at constant pressure, sound speed of seawater, and freezing-point temperature of seawater. Details are given in the cited manual.
Of special note is the use of Absolute Salinity, expressed in SI units, in the Gibbs function. This quantity is derived from the measurements underlying Practical Salinity in combination with other measurements and correlations. It is recommended by IOC that Practical Salinity remain the standard quantity for storing in national databases.
Calculators of Water Properties
Several calculators are published on the National Physical Laboratory website [www.npl.co.uk]. Of immediate relevance are the following.
Speed of sound in pure water [http://resource.npl.co.uk/acoustics/techguides/soundpurewater/]
Speed of sound in seawater [http://resource.npl.co.uk/acoustics/techguides/soundseawater/]
Absorption of sound in water [http://resource.npl.co.uk/acoustics/techguides/seaabsorption/]
Detailed source references are given on the associated webpages. Parameters to be specified by the user include temperature, pressure, salinity, and frequency, as appropriate. Limits of applicability fo the respective models are given.
Quality Assurance of Real-Time Ocean Data (QARTOD)
QARTOD is an endeavor by the U.S. Integrated Ocean Observing System (IOOS) Program Office to establish quality-assurance and quality-control procedures for a number of oceanographic variables.
At present, a total of 26 core variables have been identified as “required to detect and/or predict changes in a maximum number of phenomena of interest to user groups” [http://www.iooc.us/ocean-observations/variables/]. The U.S. IOOS core variables are: Acidity, Bathymetry, Bottom Character, Colored Dissolved Organic Matter, Contaminants, Dissolved Nutrients, Dissolved Oxygen, Fish Abundance, Fish Species, Heat Flux, Ice Distribution, Ocean Color, Optical Properties, Partial Pressure of CO2, Pathogens, Phytoplankton Species, Salinity, Sea Level, Stream Flow, Surface Currents, Surface Waves, Temperature, Total Suspended Matter, Wind Speed and Direction, Zooplankton Abundance, and Zooplankton Species.
The following website is a source of information on QARTOD. This website provides links to manuals that are available for free download. Examples include manuals on the real-time quality control of: dissolved nutrients observations, dissolved oxygen observations in coastal oceans, in-situ current observations, in-situ temperature and salinity data, in-situ surface wave data, ocean optics data, water level data, wind data.
Marine Environmental Data and Information Network (MEDIN)
Marine Environmental Data and Information Network (MEDIN) [http://www.oceannet.org/] is a partnership of UK organisations, both public and private, which aim to improve access to marine data. Data types include bathymetry, human impact, marine archaeology, marine biodiversity, marine chemistry, marine geology and geophysics, and physical oceanography. Data guidelines are provided under each of these topics, which are summarised here.
Bathymetry:
Bathymetric data, tidal elevation data, sidescan sonar data.
Human Impact:
Archiving of digital images, marine recreation data, litter data, underwater noise data.
Marine archaeology:
Archiving of digital images.
Marine biodiversity:
Ad-hoc sightings; archiving of digital images; species and benthos data by grab or core; photo-identification of cetaceans; net, pot, and trap data; species and benthos data by trawl or dredge; video survey data on species and benthos; moored oceanographic instrument data; data collected by underway oceanographic sampling; oceanographic vertical profile data; shellfish stock assessment data; water sampling.
Marine chemistry:
Archiving of digital images, moored oceanographic instrument data, data collected by oceanographic sampling, oceanographic vertical profiling data, data on water samples.
Marine geology and geophysics:
Archiving of digital images, marine gravimeter/gravity data, magnetometer/magnetic gradiometer data, seismic data, bathymetric data, species and benthos data by grab or core, sampled sediment and rock characteristics, sidescan sonar data.
Physical oceanography:
Archiving of digital images, moored oceanographic instrument data, data collected by underway oceanographic sampling, oceanographic vertical profile data, ADCP data, recordings of underwater noise, tidal elevation data.
Associated guidelines are available at the website provided above.
The Global Ocean Ship-Based Hydrographic Investigation Program (GO-SHIP)
The Global Ocean Ship-Based Hydrographic Investigation Program, abbreviated GO-SHIP, aims ‘to develop a globally coordinated network of sustained hydrographic sections as part of the global ocean/climate observing system’ [http://www.go-ship.org/index.html]. Its principal areas of interest are the carbon cycle, hydrography, marine biogeochemistry and ecosystems, and physical oceanography.
Guidelines on the collections of hydrographic data are provided in the ‘GO-SHIP Repeat Hydrography Manual: A Collection of Expert Reports and Guidelines’, IOCCP Report No. 14, ICPO Publication Series No. 134, Version 1 (2010) [http://www.go-ship.org/HydroMan.html]. Each section is available for free download at the associated links.
Additional manuals and guides are provided from the Documents webpage. These include:
GO-SHIP Repeat Hydrography Manual;
WOCE Hydrographic Program Manual;
Guide to Best Practices for Ocean CO2 Measurements (2008);
Ship of Opportunity Operations Guide (thermosalinograph installation and maintenance, XBTs, data transmission for underway variables, and like) (1999);
NOAA AOML Thermosalinograph Manual (2009);
Ocean CO2 Reference Material Program; and
Dissolved Organic Carbon CRMs.
Each of these is available for free download.
A bibliography is also provided at the webpage [http://www.go-ship.org/Bib.html].
Permanent Service for Mean Sea Level (PSMSL)
Permanent Service for Mean Sea Level is the global data bank for long-term sea level change information from tide gauges and bottom pressure recorders [http://www.psmsl.org/]. Detailed information is given about sea level measurement, as in the IOC Manuals and Guides No. 14. In Volume I [http://www.psmsl.org/train_and_info/training/manuals/ioc_14i.pdf], guidelines are given for measurement, as well as checking procedures. Volume 1, as well as Volumes II-IV, are available for free download at the given webpage.
Offshore Oil and Gas
Offshore Oil and Gas Industry Standards
The principal source of international standards used by the offshore oil and gas exploration and production industry, as well as the onshore oil and gas industry, are the following:
International Organisation for Standards (ISO)
http://www.iso.org/iso/home.html (last viewed 29 July 2013)
International Electrotechnical Commission (IEC)
http://www.iec.ch/ (last viewed 29 July 2013).
The corresponding standards used by the oil and gas industry are listed in the following:
“Catalogue of international standards used in the petroleum and natural gas industries,” International Association of Oil & Gas Producers, Report no. 362 (February 2012), 129 pp.
This is available for free download at: http://www.ogp.org.uk/pubs/362.pdf (last viewed 29 July 2013).
Several examples of ISO Technical Committees (TCs) whose standards are used by the oil and gas industry are listed together with the number of standards used by the industry, in parentheses.
TC8 Ships & marine technology (10)
TC12 Quantities, units, symbols, conversion factors (19)
TC43 Acoustics (67)
TC67 Materials, equipment & offshore structures for petroleum & natural gas industries (171)
TC69 Applications of statistical methods (15)
TC147 Water quality (3)
TC156 Corrosion of metals and alloys (12)
TC164 Mechanical testing of metals (23)
Several examples of IEC Technical Committees (TCs) whose standards are used by the oil and gas industry are listed together with the number of standards used by the industry, in parentheses.
TC18 Electrical installations of ships and of mobile and fixed offshore units (19)
TC29 Electroacoustics (8)
TC77 Electromagnetic compatibility (37)
There are also joint ISO – IEC standards, as in the case of information technology, of which 45 are listed as being used by the oil and gas industry.
Underwater Acoustics
Overview of Underwater Acoustics Standards
At the international level, there is a key standard covering the calibration of hydrophones:
IEC60565:2006, Underwater acoustics - Hydrophones - Calibration in the frequency range 0.01 Hz to 1 MHz, IEC 60565-2006.
This standard has comprehensive coverage of techniques for calibration of hydrophones in free-field conditions, and at low-frequencies in specialised couplers. It is currently under revision by IEC Technical Committee 87 (TC87) – Working Group 15 (WG15) and is likely to be divided into two parts: one for free-field techniques and one for low-frequency pressure calibration techniques.
ISO Technical Committee 43, Sub-Committee 3 (ISO TC43 SC3) covers underwater acoustics, and has produced a Publically Available Specification (PAS) covering the topic of measurement of ship noise in deep water:
ISO/PAS 17208-1:2012, Acoustics - Quantities and procedures for description and measurement of underwater sound from ships. Part 1: General requirements for measurements in deep water.
There are a number of relevant standards covering definitions and terminology produced by IEC and ISO. Four relevant standards are:
IEC 60050:1994, International Electrotechnical Vocabulary, part 801: Acoustics Electroacoustics, (section 801-32 covers terms for underwater acoustics).
IEC 60260:1995, Electroacoustics – Octave-band and fractional-octave band filters.
ISO 80000-8: 2007, Quantities and units - part 8: Acoustics.
ISO/TR 25417:2007, Acoustics - Definitions of basic quantities and terms.
All of the above standards are available from either the ISO web-store at: http://www.iso.org/iso/home.html or the IEC web-store at: http://www.iec.ch/
In the USA, ANSI has produced two standards relevant to underwater acoustic measurement:
ANSI S12.64-2009/Part 1, Quantities and Procedures for Description and Measurement of Underwater Sound from Ships - Part 1: General Requirements.
ANSI S1.20-2012, Procedures for Calibration of Underwater Electroacoustic Transducers.
ANSI S12.64 was the document upon which ISO/PAS 17208-1:2012 was based, and ANSI S1.20 provides a broader coverage than IEC 60565 as it considers the calibration of other types of electroacoustic transducers (not just hydrophones). ANSI S1.20 also provides information on a standard-target method for calibrating active sonars. These documents are available at: http://webstore.ansi.org.
Active Sonar Calibration Standards
Definitions: A sonar, or sonar system, may be defined as a system consisting of an electroacoustic transducer and a package of electronics to control the transmission and/or reception of sound. The transducer converts an electrical signal to acoustic vibrations in the contact or immersion medium, e.g., seafloor or water column. The transducer acts reciprocally, converting acoustic vibrations to an electrical signal. A hydrophone may describe any transducer used underwater, but sometimes has the narrower meaning of a transducer used only to receive underwater sound. In all cases when the subject is transducer calibration, an accompanying set of electronics to control transmission and/or reception is presumed, corresponding to an active or passive sonar system, respectively.
Performance characteristics: A sonar calibration is undertaken to determine performance characteristics of the system. For transducers used in reception, as well as passive sonar systems, a major quantity of interest is the free-field voltage sensitivity. For transducers used in transmission, major quantities of interest are the transmitting current response and transmitting voltage response. For a sonar system used in the traditional active mode to transmit sound and receive echoes resulting from its transmissions, the combined transmitting and receiving response is typically sufficient. In all cases, the directional response of transducers and sonars in transmission and/or reception is typically also of interest.
Standard procedures for calibrating electroacoustic transducers used in transmission or in reception are presented in the following standard:
ANSI/ASA S1.20-2012, “American National Standard: Procedures for calibration of underwater electroacoustic transducers” (Acoustical Society of America, Melville, NY, 2012).
This is available at http://webstore.ansi.org/RecordDetail.aspx?sku=ANSI%2fASA+S1.20-2012 (last viewed 29 July 2013).
Principles for calibrating active sonars used for echo measurement are presented in Appendix F of the same reference. This is the standard-target method developed initially for calibrating scientific echo sounders at sea (Foote 1982) and wideband echo measurements in a laboratory tank (Dragonette et al. 1981). Guidelines for calibrating scientific echo sounders by the standard-target method are presented in:
- G. Foote, H. P. Knudsen, G. Vestnes, D. N. MacLennan, and E. J. Simmonds, “Calibration of acoustic instruments for fish density estimation: A practical guide,” ICES Coop. Res. Rep., 144, (1987), 69 pp.
This is available for free download at: http://www.ices.dk/sites/pub/Publication%20Reports/Cooperative%20Research%20Report%20%28CRR%29/crr144/crr144.pdf(last viewed 29 July 2013).
The method has been extended to a variety of echo sounder systems, including those of a multiple-frequency echo sounder system (Vagle et al. 1996) and an acoustic backscattering sensor (ABS) (Foote and Martini 2010), among others.
The method has also been extended to calibration of multibeam sonars that provide the water-column signal (Foote et al. 2005). The method is also being used to calibrate parametric sonars (Westervelt 1963) of a particular type ordinarily used for profiling the sub-bottom (Dybedal 1993). Significantly, the difference frequency of this system is in the low-kilohertz band. Applications of the standard-target method span the total frequency range from 1 kHz to more than 1 MHz.
Range compensation: It is presumed that gain within the receiver electronics is controlled according to a quantitative function, formerly called time-varied gain, but now more generally called range compensation. Readily accessible references on this subject include the following: Medwin and Clay (1998), Foote (2012).
References:
L. R. Dragonette, S. K. Numrich, and L. J. Flax, “Calibration technique for acoustic scattering measurements,” J. Acoust. Soc. Am. 69, 1186–1189 (1981).
- Dybedal, “TOPAS: parametric end-fire array used in offshore applications,” in Advances in Nonlinear Acoustics, edited by H. Hobæk (World Scientific, Singapore, 1993), pp. 264-275.
- G. Foote, “Optimizing copper spheres for precision calibration of hydroacoustic equipment,” J. Acoust. Soc. Am. 71, 742-747 (1982).
- G. Foote, “Range compensation for backscattering measurements in the difference-frequency nearfield of a parametric sonar. J. Acoust. Soc. Am. 131, 3698-3709 (2012).
- G. Foote and M. A. Martini, “Standard-target calibration of an acoustic backscatter system,” in Oceans 2010 MTS/IEEE Seattle Conference Proceedings [doi: 10.1109/OCEANS.2010.5664362], 5 pp.
- G. Foote, D. Chu, T. R. Hammar, K. C. Baldwin, L. A. Mayer, L. C. Hufnagle, Jr., and J. M. Jech, “Protocols for calibrating multibeam sonar,” J. Acoust. Soc. Am. 117, 2013-2027 (2005).
- Medwin and C. S. Clay, Fundamentals of Acoustical Oceanography (Academic Press, Boston, 1998), 712 pp.
- Vagle, K. G. Foote, M. V. Trevorrow, and D. M. Farmer, “A technique for calibration of monostatic echosounder systems,” IEEE J. Oceanic Eng. 21, 298-304 (1996).
- J. Westervelt, “Parametric acoustic array,” J. Acoust. Soc. Am. 35, 535–537 (1963).
Hydrophone Calibration Standards
A number of field quantities may be used to describe a sound wave, but the most common is sound pressure. A hydrophone is a device used underwater to measure sound pressure. Hydrophones respond directly to sound pressure, as do the hearing organs of many species. It is noted that many aquatic species are also sensitive to particle motion.
The unit of sound pressure, as defined by the International System of Units (S.I.) [1], is the pascal (Pa), which is equivalent to one newton per metre squared, or N/m2.
Primary calibration methods for a measurement hydrophone are absolute calibration methods which realise the acoustic pascal in the most accurate manner available. This is most often achieved using calibration methods which depend on the principle of reciprocity.
The primary method for free-field calibration of hydrophones at kilohertz frequencies is that of three-transducer spherical-wave reciprocity [2]. This classic method requires the use of three hydrophones, at least one of which must be a reciprocal transducer; that is, its transmitting and receiving sensitivities are related by a constant factor. The hydrophones are paired off in three measurement stages, during each of which one device is used as a transmitter and the other as a receiver. For each pair of hydrophones, a calculation is made of the ratio of the voltage across the terminals of the receiving device to the current driving the transmitting device. Using the reciprocity principle as applied to the reciprocal hydrophone, the sensitivity of any one of the hydrophones can be determined from the purely electrical measurements outlined above. The achievable uncertainty for a hydrophone sensitivity using this method is typically 0.5 dB expressed at a 95% confidence level. At frequencies lower than 1 kHz, the reciprocity calibration method is typically applied to measurements made in a small chamber with dimensions smaller than the acoustic wavelength. This method is described as the coupler reciprocity method [2].
Such primary calibration methods have successfully provided primary calibration standards for many decades, but the methods have some weaknesses, particularly their dependence on the performance of a specific artefact (the reciprocal transducer). More recently, research has focused on novel calibration methods using optical techniques to realize the acoustic pascal [3], and these show considerable promise for the provision of standards into the 21st century.
Validation of the primary standard for hydrophone calibration
Whilst traceability of measurements to primary standards provides some quality assurance, there remains the question of how to determine that the primary standard itself is accurate. Since it provides the benchmark for secondary and tertiary calibrations, the primary standard cannot simply be compared to a method that is lower down the standards hierarchy. Instead, an assessment of the absolute accuracy must be made, which can be achieved in three ways:
- systematic study of the uncertainties in the primary standard calibration method. This may involve performing experiments to determine the size of the uncertainty contributions, and span many years of study [4];
- where possible, a comparison is made with another independent absolute calibration method, preferably one based on a different physical principle (and therefore with few common sources of uncertainty) [5];
- comparisons are conducted with the NMIs in different countries. Such comparisons help to harmonise standards across national boundaries and can lead to the discovery of previously unknown sources of error [6].
Figure 1 shows the results of a hydrophone calibration by two independent methods, namely free-field reciprocity and optical interferometry. The close agreement between the results (within the uncertainties of both methods – error bars not shown) illustrates the value of such comparisons in providing further confidence and validation. Note that the two methods are based on completely different physical principles and share few sources of uncertainty [3,5].
Figure 1. Comparison of calibration result for a B&K8103 hydrophone by free-field reciprocity (squares) and high-frequency optical interferometry (circles). The plot is reproduced from [5], with permission.
Figure 2 shows some of the results of the Key Comparison of hydrophone calibration organised by the Consultative Committee for Acoustics, Ultrasound and Vibration (CCAUV), which involved the NMIs of seven countries calibrating the same hydrophones [6]. The participating countries in Key Comparison CCAUV.W-K1 were UK, Germany, USA, Russia, China, Canada, and South Africa. The results are available in full at: www.bipm.org.
Figure 2. Degree of equivalence, with the uncertainties, obtained through a comparison of hydrophone calibration results of UK, Germany, USA, Russia, China, Canada and South Africa from Key Comparison CCAUV.W-K1. The plot is reproduced from [6], with permission.
The results may be expressed in terms of the degree of equivalence between the different countries along with the uncertainties. This type of exercise provides greater confidence in the individual results and allows for mutual recognition of calibrations undertaken in different countries.
Specification standards for hydrophone calibration
At the international level, there is a key standard covering the calibration of hydrophones:
IEC 60565:2006, Underwater acoustics - Hydrophones - Calibration in the frequency range 0.01 Hz to 1 MHz, IEC 60565-2006.
This standard has comprehensive coverage of techniques for calibration of hydrophones in free-field conditions, and at low-frequencies in specialised couplers. It is currently under revision by IEC Technical Committee 87 (TC87) – Working Group 15 (WG15) and is likely to be divided into two parts: one for free-field techniques and one for low-frequency pressure calibration techniques. This standard is available from the IEC web-store at: http://www.iec.ch/.
In the USA, ANSI has a similar standard relating to the calibration of hydrophones:
ANSI S1.20-2012, Procedures for Calibration of Underwater Electroacoustic Transducers.
ANSI S1.20 provides a broader coverage than IEC 60565 as it considers the calibration of other types of electroacoustic transducers (not just hydrophones). ANSI S1.20 also provides information on a standard-target method for calibrating active sonars. This document is available at: http://webstore.ansi.org.
Hydrophone usage guidelines
There are practical considerations about electrical loading, mounting, and wetting, for which guidelines have been prepared. Links are provided in the following.
Hydrophone electrical loading corrections [http://resource.npl.co.uk/acoustics/techguides/loading/]
Hydrophone mounting [http://www.npl.co.uk/acoustics/underwater-acoustics/research/hydrophone-mounting]
Hydrophone wetting [http://www.npl.co.uk/acoustics/underwater-acoustics/research/hydrophone-wetting]
References
[1] The International System of Units (SI), Bureau International des Poids et Mesures (BIPM), Paris. Brochure available from www.bipm.org
[2] R. J. Bobber, Underwater Electroacoustic Measurements, Naval Research Laboratory, Washington, DC, 1970 [Peninsula Press, USA, (2nd edition), 1989].
[3] P. D. Theobald, S. P. Robinson, A. D. Thompson, R.C. Preston, P. A. Lepper and W. Yuebing, “Technique for the calibration of hydrophones in the frequency range 10 to 600 kHz using a heterodyne interferometer and an acoustically compliant membrane,” J. Acoust. Soc. Am. 118(5), pp. 3110-3116, 2005.
[4] P. M. Harris, G. Hayman, S. Robinson and I. Smith. “Uncertainty evaluation of an underwater acoustic transducer by the method of free-field reciprocity,” NPL Report MS9, October 2010. Available from www.npl.co.uk
[5] S. P. Robinson and P. D. Theobald, “Validation of primary hydrophone calibrations by inter-laboratory comparisons and by independent calibration methods,” J. Acoust. Soc. Am., 123, p3346, 2008. Full paper reproduced in Proceedings of the 9th European Conference on Underwater Acoustics (ECUA2008), ed. M. Zakaria, pub. Société Française d’Acoustique, vol 1, pp125-130, July 2008.
[6] S. P. Robinson, P. M. Harris, J. Ablitt, G. Hayman, A. Thompson, A. L. Van Buren, J. F. Zalesak, R. M. Drake, A. E. Isaev, A. M. Enyakov, C. Purcell, H. Zhu, Y. Wang, Y. Zhang, P. Botha, and D. Krüger, “An international key comparison of free-field hydrophone calibrations in the frequency range 1 kHz to 500 kHz,” J. Acoust. Soc. Am., vol 120 (3), p 1366 – 1373, 2006.
Standards for Underwater Noise Measurement
The measurement of underwater noise levels in the marine environment is a topic of increasing importance, driven by the concern that there are increasing numbers of anthropogenic noise sources in oceans and the effects of the radiated underwater noise on marine fauna. There is also a need to perform underwater noise measurements to conform to international, national, and local regulatory requirements.
There can be substantial variation in the methods used to measure underwater noise, and the metrics used for reporting the noise levels can vary depending on the application, which can result in ambiguity.
Recognising the need for standardization of underwater noise measurement, work is being carried out under the auspices of the International Organization for Standardisation (ISO), within ISO Technical Committee 43, Sub-committee 3 (ISO TC43 SC3) which has the title “Underwater Acoustics”. Within ISO TC3 SC3, there are currently two active working groups (WG), of direct relevance to underwater noise measurement, with the following remits:
WG1: Measurement of underwater sound from ships;
WG3: Measurement of radiated noise from marine pile driving.
A third working group, WG2: Underwater acoustical terminology, is developing definitions that include concepts and quantities in underwater noise.
As a parallel activity, but independent to that of ISO TC43 SC3, a Good Practice Guide for Underwater Noise Measurement has been produced by the National Physical Laboratory (NPL), United Kingdom, describing best practice for in-situ measurement of underwater noise, data processing, and reporting of the measurements using appropriate metrics.
This Guide addresses the need for a common approach and the desire to promote best practice. It is designed for those making in-situ measurements of underwater noise, for example, consultants, offshore developers, oil and gas companies, and developers of marine renewable energy, as well as regulators wishing to base their findings on a firm scientific foundation.
A copy of this Good Practice Guide can be downloaded here:
www.npl.co.uk/publications/guides/good-practice-guide-for-underwater-noise-measurement
The work to prepare this Good Practice Guide was funded by National Measurement Office (an executive agency of the Department for Business, Innovation and Skills), Marine Scotland, and The Crown Estate.
Other guidance documents relating to the measurement and reporting of underwater noise are:
- A. Ainslie, “Standard for measurement and monitoring of underwater noise, Part I: physical quantities and their units,” TNO Report TNO-DV 2011 C235 (2011).
- A. F. de Jong, M. A. Ainslie, and G. Blacquière, “Standard for measurement and monitoring of underwater noise, Part II: procedures for measuring underwater noise in connection with offshore wind farm licensing,” TNO Report TNO-DV 2011 C251 (2011).
- Müller and C. Zerbs, “Offshore wind farms - Measuring instruction for underwater sound monitoring,” Application instructions for Bundesamt für Seeschifffahrt und Hydrographie, Germany, October 2011.
Published standards of relevance to underwater noise measurement also include:
ANSI/ASA S12.64-2009/Part 1, 2009, Quantities and Procedures for Description and Measurement of Underwater Sound from Ships - Part 1: General Requirements, American National Standard Institute, USA, 2009.
ANSI/ASA S1.20-2012, Procedures for Calibration of Underwater Electroacoustic Transducers, American National Standard Institute, USA, 2012.
IEC 61260:1995, Electroacoustics - Octave-band and fractional-octave-band filters, International Electrotechnical Commission, Geneva, Switzerland, 1996.
IEC 60565:2006, Underwater acoustics-Hydrophones - Calibration in the frequency range 0.01 Hz to 1 MHz, IEC 60565 - 2006 (EN 60565: 2007, BS60565:2007), International Electrotechnical Commission, Geneva, 2006.
IEC 60050:1994, International Electrotechnical Vocabulary, part 801: Acoustics and Electroacoustics, (section 801-32 covers terms for underwater acoustics), International Electrotechnical Commission (IEC), Geneva, 1994.
ISO1996-1:2006, Acoustics – Description, measurement and assessment of environmental noise – Part 1: Basic quantities and assessment procedures. International Organization for Standardization, Geneva, 2006.
ISO 80000-8:2007, Quantities and units – part 8: Acoustics, International Organization for Standardisation, Geneva, 2007.
ISO/TR 25417:2007, Acoustics — Definitions of basic quantities and terms. International Organization for Standardisation (ISO), Geneva, 2007.
ISO/PAS 17208-1:2012, Acoustics — Quantities and procedures for description and measurement of underwater sound from ships. Part 1: General requirements for measurements in deep water, International Organization for Standardisation, Geneva, 2012.
JCGM 100:2008, Evaluation of measurement data – Guide to the Expression of Uncertainty in Measurement (GUM), joint publication by BIPM, IEC, IFCC, ILAC, ISO, IUPAC, IUPAP and OIML, 2008. Available from www.bipm.org.
Current Developments in Underwater Acoustics Standards
There are a number of current developments within ISO TC43 SC3, which has four working groups actively developing standards.
WG1: Measurement of underwater sound from ships
Under the leadership of the USA, this working group is working to produce a series of standards related to the measurement of underwater noise from ships. The Publically Available Specification published in 2012, “ISO/PAS 17208-1:2012 – Acoustics – Quantities and procedures for description and measurement of underwater sound from ships – Part 1: General requirements for measurements in deep water,” has now been superseded by a published standard, “ISO 17208-1:2016 – Acoustics – Quantities and procedures for description and measurement of underwater sound from ships – Part 1: Requirements for precision measurements in deep water used for comparison purposes.”
The Committee Draft ISO 17208-2 is currently being split into two parts, the first being ISO 17208-2, “Underwater acoustics - Quantities and procedures for description and measurement of underwater noise from ships - Part 2.” The second part intends to address primarily the determination of source level from deep water measurements. The proposed title of the projected standard, ISO 17208-3, is “Underwater acoustics - Quantities and procedures for description and measurement of underwater noise from ships - Part 3.”
WG2: Underwater acoustical terminology
Led by the Netherlands, WG2 has been developing a standard for terminology and definitions of quantities used in underwater acoustics. The Final Draft International Standard is being prepared, with designation ISO 18405, “Underwater acoustics – Terminology.”
WG3: Measurement of radiated noise from marine pile driving
Led by the UK, WG3 is developing a standard for measurement of radiated noise from marine percussive pile driving. The Draft International Standard ISO 18406, “Underwater acoustics — Measurement of radiated underwater sound from percussive pile driving,” is currently being modified and will be developed into a Final Draft International Standard for national balloting.
WG4: Standard-target method of calibrating active sonars
This WG began work in 2015 under leadership of the USA. WG4 is developing a standard with the title “Underwater acoustics - Standard-target method of calibrating active sonars for imaging and measuring scattering,” with advance designation ISO 20073.
OES Interoperability Standards Initiative for the Marine Environment
The IEEE Oceanic Engineering Society has been pursuing a project called the Interoperability Standards Initiative for the Marine Environment. This initiative aims to define the need for interoperability with respect to sensors used in systems in the marine environment. Both hardware and software are subjects. It also aims to formulate a specific plan of action to address that need, principally through the establishment of standards. The expected impact is that of standardization in the design and manufacture of sensors, with direct benefits to the manufacturer and user in enabling easier, more stable, and efficient connections and communications when assembling systems, and processing and analyzing derived sensor data.
A thematic session on “Interoperability standards for the marine environment” was held during Sea Tech Week in Brest, France, 11-13 October, specifically during “A Connected Ocean” Conference (ACO 2016), 11-12 Oct. The intention was to convene a group of experts and other interested parties to summarize and review the state of the art in interoperability standards: to share current knowledge with ocean engineers and managers who are charged with the design of future ocean networks and observatories. Papers were given by Tom O'Reilly, Jean Francois Rolin, Catherine Satra Le Bris, Joaquin del Rio, Hozi Bao Lam, Janet Fredericks, Gilbert Maudire, and Fadi Obeid. Interests and opportunities were discussed in conclusion.
Ocean engineers are aware of the importance and value of interoperability standards for marine applications, and these standards are being adopted in a number of European Union projects. The value, of course, transcends any one geographical region. The work of the NSF-supported OceanObs Research Coordination Network, which is currently led by OES members, is contributing to the wider adoption of interoperability standards.
Standards Board Resolutions
Meet the Technical Committee
Christoph Waldmann | University of Bremen, MARUM, Germany (Chair) |
Steve Holt | KBR, Inc., USA (Vice Chair) |
Fausto Ferreira | LABUST, Faculty of Electrical Engineering and Computing, University of Zagreb, Croatia (Secretary) |
Tom O’Reilly | Monterey Bay Aquarium Research Institute, USA |
Fan Jiang | National Center of Ocean Standards and Metrology of SOA, Tian Jin, China |
Xianbo Xiang | Lab of Advanced Robotic Marine Systems, School of Naval Architecture and Ocean Engineering, Wuhan, China |
Bob McCummins Jr. | LEIDOS, Reston, VA, USA |
Shashikant Patil | Electronics & Telecommunication Engineering Department, Mukesh Patel School of Technology Management & Engineering, Shirpur, India |
Tomorou Yamada | Japan |
Sigmund Kluckner | Consultant, Vienna, Austria |
Marinna Martini | NOAA Fisheries, Woods Hole, MA, USA |
Jay Pearlman | Four Bridges, Port Angeles, WA, USA |
Venugopalan Pallayil | National University of Singapore |
Rene Garello | Past-President OES, France |
Michael A. Enright | Quantum Dimensions Inc., USA |